Multi-scale, multi-physics modelling of nickel nanohoneycomb actuator
Motivation: Commercial softwares such as ANSYS or Abaqus are readily available for simulating the deflection or stress distribution in common materials under different loading conditions. However, these softwares are insufficient to predict the deflection of nanoporous actuators, since the macroscopic strain is due to the change in length happening at different length scales governed by different mechanisms. We have to start from scratch at the atomic scale, and move up the length scale step by step as we calculate the macroscopic strain, which is beyond the scope of most commerical softwares.
​
Currently, there exists some studies which employs ab initio calculation and multi-scale simulations to predict the charge-induced deformation of nanoporous gold actuators in vacuum. However, this method is not sufficient to describe actuation of nanoporous Ni since its actuation mechanism is more complex. Actuation in nanoporous Au/Pt can be modeled as electrocapillarity [2] at a clean metal surface, where there exist a relationship between the surface stress (and thus strain) of the material and its surface charge density. While the change in surface charge density also contributes to a small part of strain for nanoporous nickel actuator, we discovered through our previous studies that redox reaction at the surface layer dominates most of the macroscopic strain. This surface layer consisted of nickel hydroxide (Ni(OH)2), formed under oxidation in alkaline electrolytes during actuation, is known to undergo redox reaction Ni(OH)2<-> NiOOH when an alternating voltage of 0V/0.5V (with respect to saturated calomel electrode, SCE) is applied to actuator. The oxidation of Ni(OH)2 to NiOOH is accompanied by lattice volume reduction which give rises to dimensional changes. As could be seen from the description above, the actuation mechanism of nanoporous nickel actuator involves surface electrochemistry, charge transfer, and mechanics. Ab initio approach makes the modelling work practically intractable and insuitable to be applied to nanoporous nickel actuators.
​
Method: We adopted a multi-scale, multi-physics modelling approach to predict the end-deflection of a cantilever Ni nanohoneyconb actuator. We also compared the simulated deflections with the experimental values obtained from previous research.
At the atomic scale, molecular dynamics (MD) based on reactive force-field potentials (ReaxFF) was performed suing a commercial software LAMMPS to simulate the building up of surface stress along the Ni/water interface. The calculated surface stress is then used as input in a meso-scale finite-element model to compute the actuating stress set up in a single hexagonal unit cell of the Ni nanohoneycomb structure. The single-unit actuating stress is eventually used in a continuum FE model at the macroscopic scale, to calculate the bending of an entire nanohoneycomb Ni cantilever.
​
Close prediction of deflection to experimental values: The actuation deflection of the bilayered nanohoneycomb Ni is predicted to be 41.4µm at ~0.48V vs SCE, which is in excellent agreement with the experimental value of 45-62 µm at a similar voltage vs SCE reference electrode.
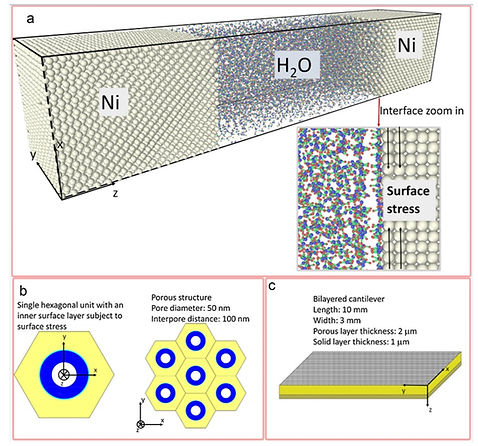
1. Molecular dynamics modelling at atomic scale
2. Meso-scale stress distribution in hexagonal units of nanohoneycomb structure
3. Macro-scale deflection prediction through FEM
The multi-scale, multi-physics approach to predict end-deflection in nanohoneycomb Ni actuators
References:
[1] Zhang YQ, Ngan HW. 2017. Multi-scale, multi-physics modeling of electrochemical actuation of Ni nanohoneycomb in water. Computational Materials Science, 128:109–120.
​
[2] Kramer D, Weissmüller J. 2007. A note on surface stress and surface tension and their
interrelation via Shuttleworth's equation and the Lippmann equation. Surf Sci, 601:3042–51.

The predicted deflection using the multi-scale, multi-physics approach is indicated by the orange dashed line. It could be seen that the modeled deflections agrees well with experimental values from -0.1V ~ 0.2V.The predictions are only slightly lower than experimental data at higher potentials, but still it is in the same order of magnitude.